Table of Contents Hide
- 1 What Is Horticulture Lighting
- 2 Light Spectrum
- 3 Photosynthesis
- 4 Photomorphogenesis
- 5 Photoperiodism
- 6 Photoreceptors
- 7 Action Spectrum
- 8 Artificial Lighting in Horticultural Applications
- 9 Types of Horticulture Lighting Installations
- 10 Photosynthetically Active Radiation (PAR)
- 11 Lighting Technologies
- 12 How LED Horticulture Lighting Works
- 13 Smart Horticulture Lighting
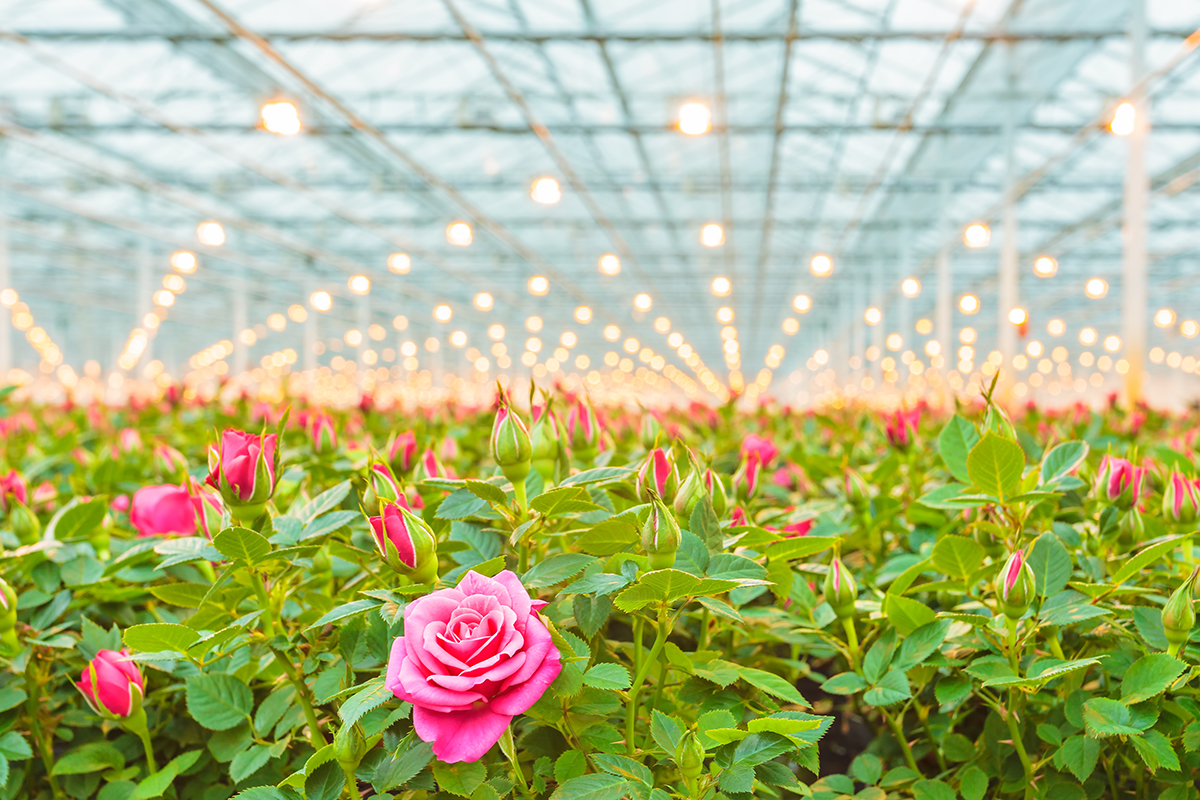
What Is Horticulture Lighting
Horticulture lighting refers to electromagnetic radiation that stimulates different types of photoreceptors in plants in order to drive photosynthesis, photomorphogenesis, photoperiodism and phototropism. All plants, including those flowering, fruiting and vegetable plants, require light, water, carbon dioxide and nutrients to survive and thrive. Light is used by plants to catalyze the chemical reaction of carbon dioxide and water thereby synthesizing carbohydrates that allow the plants to grow. Plants also have evolved to perceive light as a signal that enables long-term developmental and short-term dynamic acclimation to the environment. Plants must receive sufficient light to fuel metabolic process, drive active growth, maintain plant quality and achieve maximal yields. As the intensity and spectrum of sunlight often fluctuate, artificial light sources are used to address the needs of plant growth either as a supplementary light source to the sun or as a sole-source of photosynthetic and photomorphogenic light.Light Spectrum
The energy produced by the sun reaches the earth as electromagnetic radiation which travels in packets of energy called photons. Each photon has a characteristic energy that determines the frequency of vibration or oscillation. The distance that a photon moves during the oscillation is referred to as a wavelength and is measured in nanometers. Electromagnetic radiation spans a broad range of wavelengths - from 100 nm to about 1 mm (1,000,000 nm). A very small part of this spectrum is visible to the human eye i.e. between 380 nm and 780 nm. Electromagnetic radiation that falls in this range of wavelengths is called visible light.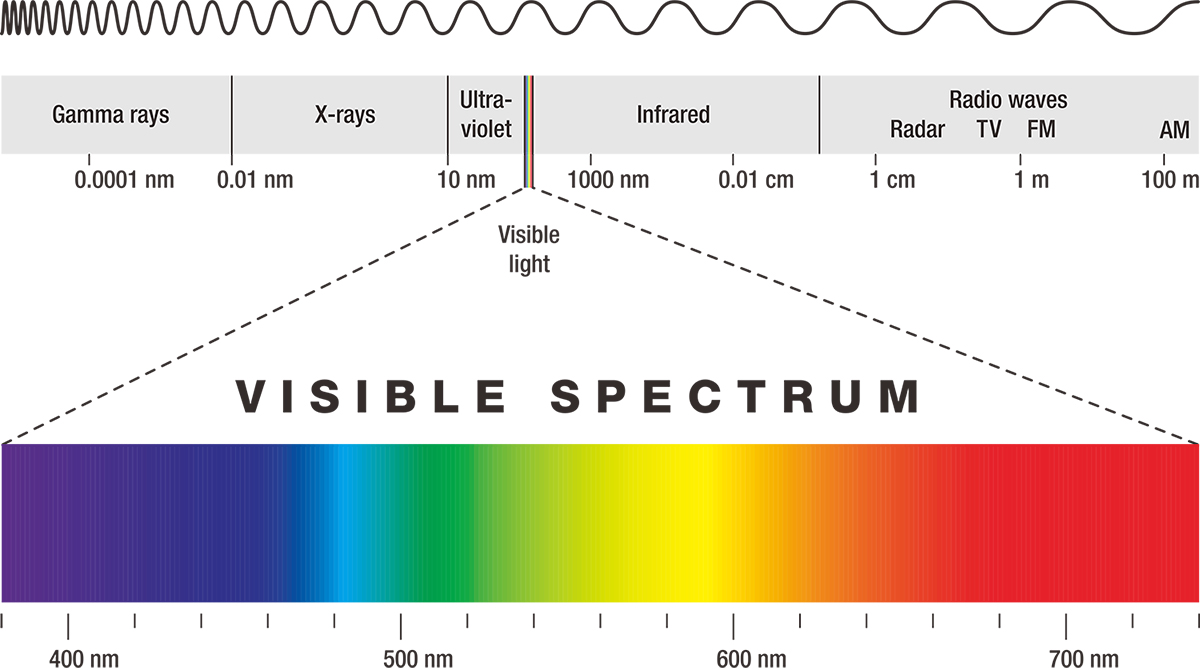
The absorption spectrums of plants are also within the visible part of electromagnetic radiation but has a relatively narrower range: 400 nm - 700 nm. While humans perceive the different visible wavelengths as different colors, plants interpret wavelengths in the active spectrum in a completely different way.
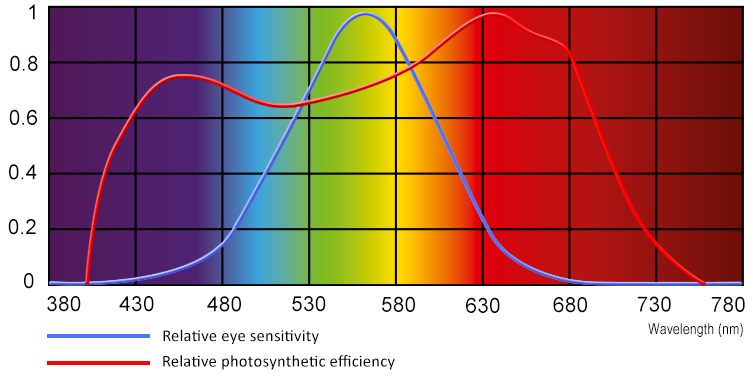
Photosynthesis
The photobiochemical process that uses light as a catalyst to drive the synthesis of glucose from carbon dioxide and water is called photosynthesis. Photosynthesis is the ultimate source of metabolic energy for all plants. The photobiochemical process can be divided into two phases: light dependent reactions (light reactions) and light-independent reactions (dark reactions). In the light reactions, energy from sunlight is harvested to drive the synthesis of adenosine triphosphate (ATP) and reduced nicotinamide adenine dinucleotide phosphate (NADPH), while releasing oxygen as a waste product. In the dark reactions (so named because they do not use light), carbon dioxide is modified by the addition of hydrogen to form glucose and ultimately other carbohydrates, proteins and fats. The assembly of carbon atoms in organic molecules requires the energy-releasing cleavage of high energy bonds of ATPs and NADPHs. In this phase, the ATP loses one of its three phosphates to become ADP (Adenosine diphosphate) and the NADPH loses one electron to become NADP+. The two phases of photosynthesis are interlinked and complimentary. The energy-depleted ADPs and 12 NADPs are restored in light reactions to their high energy forms (ATP and NADPH).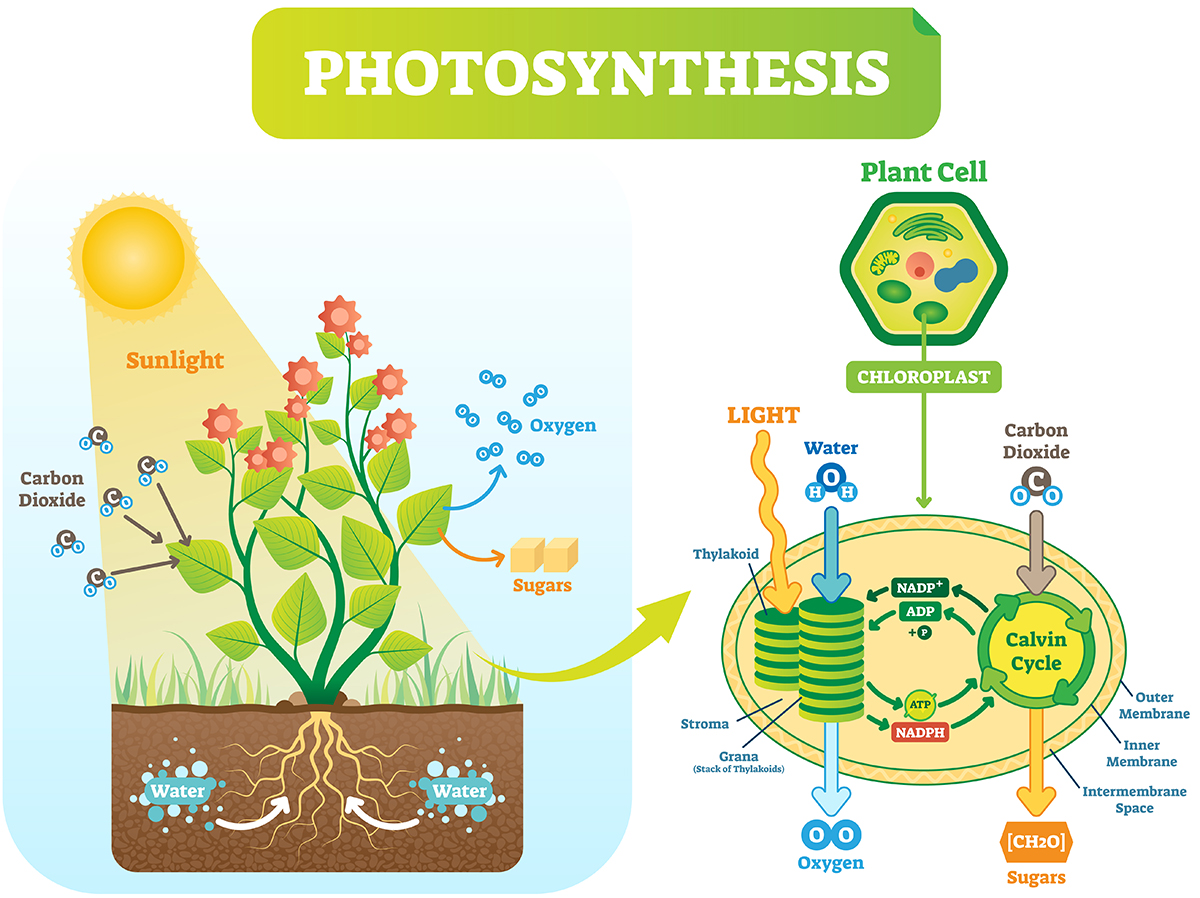
Photomorphogenesis
Apart from being used as a source of energy to photosynthesize carbohydrates required for plant growth, light is simultaneously used by plants as a source of information to modulate their growth and development to match the conditions in their environment. When exposed to light, plant tissues undergo the process of photomorphogenesis, which enables optimization of resource-use efficiency and acclimation of photosynthesis to available irradiance from germination through to the transition to flowering and fruit ripening. Photomorphogenesis describes light dependent changes in plant morphology. The photomorphogenic effects influence stem elongation, stomatal opening, leaf expansion, seed germination, phototropism (bending toward light), and photonasty which refers to the nastic movement of a plant part (such as flower opening) in response to light.Photoperiodism
The length of time a plant receives light during a 24-hour cycle is called photoperiod. Photoperiodism refers to the ability of plants to measure photoperiod to determine the season. This ability enables plants to regulate biological activities in the response to environmental rhythms of light and darkness. Plants can be categorized into short-day plants that require a long dark period, long-day plants that typically require a photoperiod of 16-18 hours, and day-neutral plants which are not affected by photoperiodism. This rhythmic characteristic of plants is utilized to photoperiodically manipulate flowering time.Photoreceptors
Plants have a sophisticated network of photoreceptors that harvest light energy to drive photosynthetic processes, or respond to changes in light conditions to regulate photomorphogenesis and photoperiodism.Chlorophylls, which reside in the thylakoids of organelles called chloroplasts, are the principal type of photoreceptors that capture energy from electromagnetic radiation for photosynthesis in plants. Chlorophylls absorb wavelengths only in the red and blue regions of the visible light spectrum. Because they reflect green wavelengths, leaves of most plants appear green and thus chlorophylls are also called pigments. Chlorophylls come in two major forms, A and B.
Chlorophyll A is the primary active pigment in photosynthesis and accounts for around 75% of wavelength absorption capacity of a chloroplast. Upon absorption of a photon, an electron in the chlorophyll A molecule is excited and is then passed to a modified form of chlorophyll called pheophytin, which passes the electron to a quinone molecule. The flow of the electron down an electron transport chain eventually causes the electron to react with NADP and change this carrier molecule from its oxidized state (NADP+) to its reduced state (NADPH). Meanwhile, photoexcitation of chlorophyll creates a proton gradient (energy gradient) across the chloroplast membrane, which is ultimately used to drive the synthesis of ATP from ADP and phosphate. Chlorophyll A has its sensitivity peaks in the red and blue ranges of the spectrum at wavelength 430 and 680 nm respectively. Chlorophyll B, which exhibits peak absorptions at 460 nm and 640 nm, does not participate in light reactions. It captures light energy and then passes it to chlorophyll A through electron spin resonance.
Accessory pigments or antenna photoreceptors absorb electromagnetic energy that chlorophyll A does not absorb. Aside from chlorophyll B (and also C, D, and E in algae and protistans), accessory pigments include the carotenoid family. Carotenoids, such as beta-carotene, lutein, zeaxanthin, antheraxanthin and violaxanthin, capture wavelengths other than only red and blue. Carotenoids are yellow, red or orange in color as they reflect the wavelengths outside of the photosynthetically active range between 450 nm to 550 nm. Carotenoids collect about 10% of the photosynthetically active light, 90% of light is harvested by chlorophyll pigments. In addition to contributing to the photosynthesis, carotenoids also offer photoprotection for plants when excessive light or UV radiation generates oxygen radicals (molecules with unpaired electrons) and causes photoinhibition. These antioxidants protect the chlorophylls from photooxidation and prevent further cellular damage by dissipating excess light as heat through an interconversion process called the xanthophyll cycle.
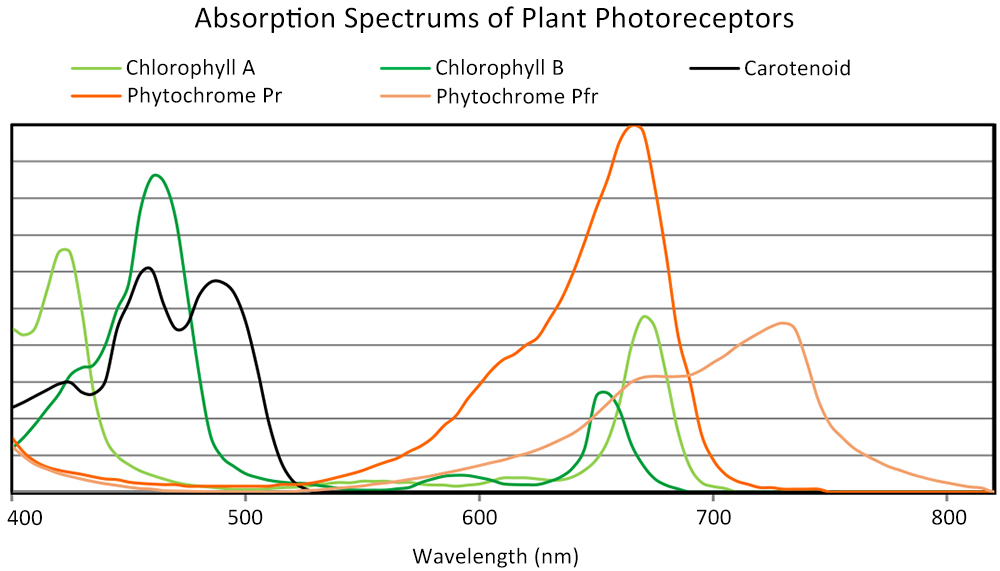
Plants have a range of non-photosynthetic photoreceptors that do not harvest light energy. Instead they perceive light as a developmental signal that enables plant growth to react to the light environment (intensity, spectrum, direction and duration of irradiance). Phytochromes, cryptochromes and phototropins are signal-transducing photoreceptors responsible for regulating plant growth based on the photomorphogenic effects of light. The phytochromes Pr and Pfr, which respond to 660 nm red light and 735 nm infrared radiation respectively, regulate a variety of processes including germination, stem elongation, apical hook straightening, leaf expansion, flowering induction, circadian rhythm entrainment, and chlorophyll biosynthesis. Cryptochromes are involved in regulating photomorphological responses, such as pigment synthesis, photoperiodism (circadian rhythm), flowering, enzyme synthesis and inhibition of hypocotyl elongation by changing gene expression. Phototropins regulate cellular processes to mediate stomatal regulation, chloroplast movement, leaf flattening, and phototropism (plant movement towards light). Cryptochromes and phototropins both function across the blue and UVA regions of the spectrum with absorption peaks at 350 nm (UVA) and 450 nm (blue).
There are non-photosynthetic and non-photomorphogenic accessory pigments in plants such as flavonoids, anthocyanins and lycopenes which are potent antioxidants. When blue (400 - 500 nm) or ultraviolet (300 - 400 nm) radiation carries a tremendous amount of energy that can cause cellular damages, resources are diverted away from the primary metabolism (growth) and the plant's secondary metabolism is triggered. In order to protect plant tissues against high energy radiation and clean up free radicals, production of flavonoids and anthocyanins scales up with incoming energy to reach an equilibrium of photoprotection. At the same time this secondary metabolic process causes leaves and flowers to have stronger colors.
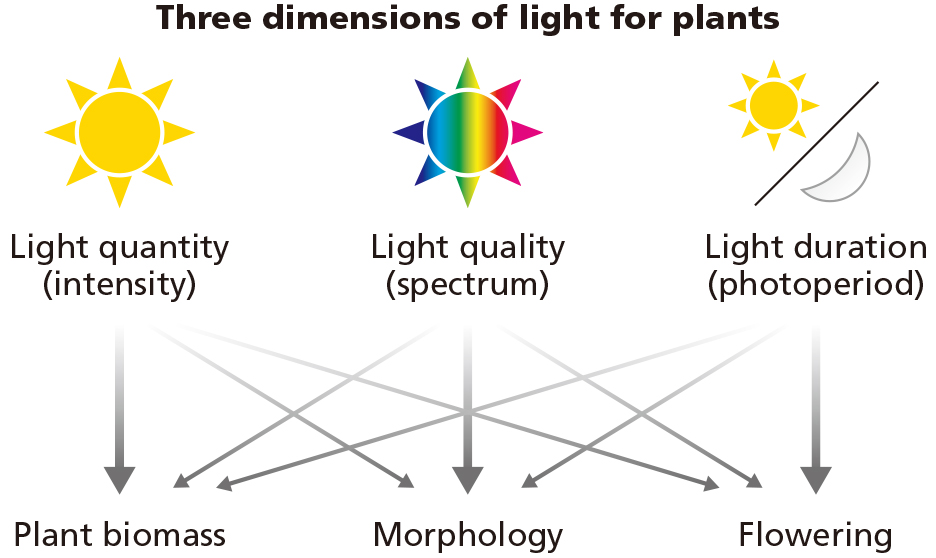
Action Spectrum
The spectrum of light strongly affects the primary metabolic processes (growth and development of leaves, stems, roots, and floral organs) and production of secondary metabolites (flavonoids, terpenes, cannabinoids). Photosynthetic chlorophylls and carotenoids, and other accessory pigments, absorb more efficiently blue and red light. However, all plants have a species-specific light preference, and photoreceptors that influence their anatomical, physiological, morphological and biochemical properties can span multiple wavelengths. For horticultural lighting, one of the major challenges is to develop a light spectrum that enables efficient activation of different photoreceptors for optimal results in all aspects of plant growth, including photosynthesis, photomorphogenesis, photoperiodism and phototropism.Red light (600 – 700 nm) offers almost twice the quantum efficiency of blue light for driving photosynthesis. Wavelengths within this range can trigger phytochrome responses related to germination (photoblasty), pigment formation, stem growth, flowering (photoperiodism), circadian rhythm entrainment, and dormancy. Red light is crucial for carbohydrate synthesis, hormone activation, improving phenolic compound concentrations to promote rooting. In general, plants exposed to more red light, in particular hyper red with its absorption peak located at 660nm, grow taller and faster than plants exposed to more blue light.
Blue light (400-500 nm) is another main contributor to photosynthesis. Blue light is also known to trigger two families of photomorphogenic photoreceptors: phototropins and cryptochromes. Wavelengths in this range have been linked to the regulation of stomata opening, chlorophyll concentration, lateral bud growth, root development, transition to flowering, enzyme synthesis, and leaf thickness. High blue irradiation cause plants to have reduced leaf internodal length, compact and bushy growth, high dry matter content and low leaf temperature (efficient transpiration). High deep blue content is needed for seedlings to start germinating and sprouting. Blue light is particularly effective at promoting stem elongation and leaf expansion. However, over exposure to the high energy blue wavelengths can inhibit plant growth.
Green light (500–600 nm) was conventionally thought to have minor importance in biology because plants reflect wavelengths in this range. However, there is strong evidence that green light can penetrate deeper into the plant canopy and, therefore, makes a unique contribution to photosynthetic carbon assimilation and biomass accumulation on both a leaf and canopy level. The absorption of green photons by anthocyanins suppresses production of superoxide, which can cause free radical damage to plant cells. Irradiation with blue light causes plants to have long petioles internodes and high leaf temperature. Green light is partially perceived by phototropins and cryptochrome. When green light is the only source it can induce a small degree of stomatal opening.
Far red light (700-800nm) is absorbed by phytochromes of the Pfr form, which has the absorption peak at 730 nm. Phytochromes are the main regulators of plant circadian rhythm and shade avoidance mechanisms. An additional channel for far red supports quick development of healthy plant biomass which enable large flower formations. Far red light works with deep red light to either delay or advance flowering based on day length perception. High far-red irradiation causes elongation of stem and petioles and allows plants to grow in a more compact form.
UV light, including UV-A (320-400 nm) and UV-B (280 - 320 nm), can cause photoinhibition of chloroplasts when applied incorrectly, resulting in reduced photosynthetic rates, low biomass production, photobleaching and death of leaves. Cryptochromes, phototropins, and members of the Zeitlupe/Adagio family can be stimulated by UV-A light. UV-B light is captured by the UVR8 photoreceptor. In small doses, both UV-B and UV-A light increase the stress tolerance of plants and cause plants to create more secondary metabolites to strengthen their defense mechanisms. Plants grown under ultraviolet radiation tend to have thick leaves and stems, and short internodes.
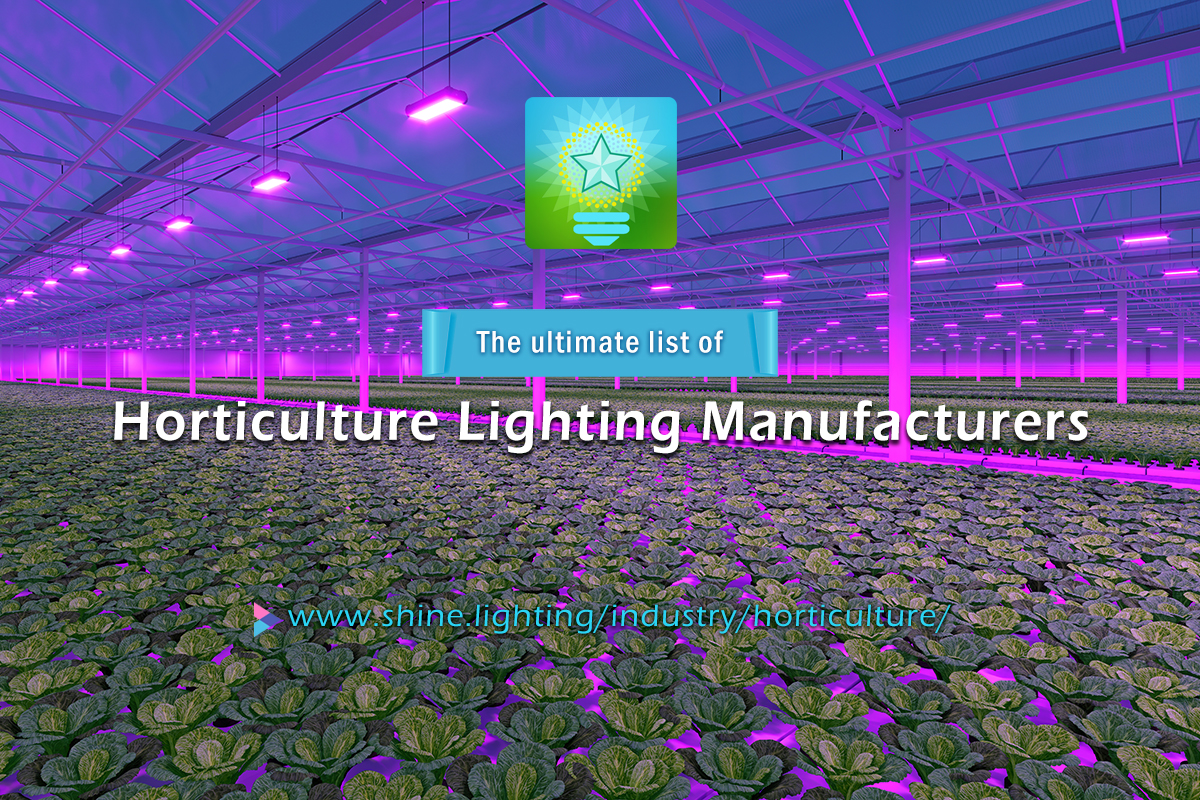
Artificial Lighting in Horticultural Applications
The individual sensitivities of plant photoreceptors open up opportunities for selective intervention in the metabolic processes and biochemical signalling pathways of plants using artificial light sources. Horticulture lighting is intended to simulate the plant-friendly spectrum of sunlight and provide photosynthetically, photomorphogenically, and photoperiodically active spectrums to support plant growth, development, and yield. By targeting various photoreceptors with select wavelengths and applying the optimum light recipe at every stage of a plant's growth, growers are able to invoke desired photosynthetic responses and morphological changes in their plants.Horticulture lighting systems play several roles in plant growth:
Supplemental lighting is typically provided in greenhouses where natural sunlight fails to provide the desired daily light integral (DLI) for propagation and transplant production during light limiting conditions (e.g. winter months in northern latitudes, cloudy conditions). Supplemental lighting not only enables enhanced photosynthesis and thereby improves growth and quality of plants, it can also be used to prolong shelf-life or to alter the biochemical properties after harvesting.
Photoperiodic lighting is used to control the flowering times of certain short-day and long-day plants such as chrysanthemum, euphorbia pulcherrima, kalanchoe, gypsophilia and carnations. To induce early or out-of-season flowering for predetermined market dates, the photoperiod of a plant is modified by extending the day length to trick the plant into behaving in the desired way. In addition to flowering induction, photoperiodic lighting is also applied in the seed germination process.
Sole-source lighting is designed for full-cycle cultivation in controlled environment facilities, multi-tier vertical farms, growth chambers and containers. This means all the light is produced by artificial light sources. It is therefore essential that the spectral composition of the artificial light is balanced for optimal plant development at different growth stages from seedling, germination, flowering, fruiting and harvesting.
Types of Horticulture Lighting Installations
Top lighting — plants are illuminated from ceiling level. This type of light installation is mostly found in greenhouses which still take advantage of light from the sun and use artificial light sources to supplement natural daylight. High ceiling mounting with wide-angle beam distribution maximizes upper-canopy photon capturing efficiency and reduces fixture density. However, top lighting systems consume a considerable amount of power in order to ensure that sufficient photosynthetically active photons reach plant canopies across a distance. Traditional light fixtures such as high pressure sodium (HPS) and metal halide (MH) lights radiate a high percentage of thermal energy that can increase plant temperature and therefore must be mounted at some minimum distance above the plants.Vertical farming — racks of plants are stacked vertically on top of each other and lighting is positioned a short distance from plants. Using controlled environment agriculture (CEA) technology, growers are able to cultivate high density crops in facilities with a low land-area footprint. In vertical farms, sunlight is not available and artificial light is the only source of light. Light fixtures for multi-layer cultivation applications must have a low profile and cannot emit infrared energy because they are mounted directly above and in close proximity of the crop. Growers have complete control over the spectrum and intensity of the sole-source lighting.
Interlighting (intracanopy lighting) — a multi-directional, and typically linear lighting solution designed to provide photosynthetically active lighting along the side or within the foliar canopy in a greenhouse. Light sources are placed in between the plants and the leaves, which prevents mutual shading and encourage previously shaded leaves to photosynthesize. As with vertical farming, intracanopy lighting requires a "cold" light source so that plants will grow safely.
Photosynthetically Active Radiation (PAR)
Electromagnetic radiation over the spectral range of 400 nm to 700 nm is called photosynthetically active radiation (PAR), as predominantly the wavelengths in this range are used by plants to drive photosynthesis. Plant grow lights are primarily designed to target this spectral range, and in some cases provide additional electromagnetic radiation to activate photoreceptors which have absorbance wavelengths outside the PAR region. A plant grow light must convert as much electrical energy as possible into PAR energy. As such, horticulture lighting systems are evaluated by their ability to stimulate photosynthesis. Rather than quantifying the photopic sensitivity by "luminous flux", radiation flux of a grow light is converted into photosynthetic photon flux (PPF) in the PAR region. Assessing interaction between radiation and plants is focused on the quantities of hotosynthetically active photons that fall on plant canopies. Listed below are the most important metrics in horticulture lighting.Photosynthetic photon flux (PPF) measures the amount of photons emitted by a light source in the PAR region, and is expressed in micromoles per meter squared per second (µmol/s). PPF tells the photon output of a light source, it however does not provide the amount of photons that land on the plants.
Photon efficacy (PPF/W), expressed in micromoles per joule (µmol/J), provides a clear picture of the energy conversion efficiency of a horticulture lighting system or a light source. This metric indicates the total number of photosynthetically active photons generated by one joule of electrical energy.
Photosynthetic photon flux density (PPFD) is a measure of photosynthetically active photons that fall on a square meter of the target area per second. The unit of this metric is expressed as "μmol/m2/s". PPFD is the most important metric with regard to lighting deployment because it provides a field measurement of the number of photons that are incident on a plant canopy. It therefore makes sense to collect this data in order to make sure that neither energy is wasted nor photosynthesis is underdriven. Every plant species has a light saturation point beyond which the rate of photosynthesis stops rising and additional light becomes wasted. The sun delivers approximately 2000 µmol of PPFD at sea level on a bright sunny day. However no plant species on earth can absorb this full quantity of photon flux. A PPFD between 400 and 800 μmol/m2/s is sufficient to drive photosynthesis in plants.
Daily light integral (DLI) quantifies the total number of photons that a plant absorbs over a 24-hour period and is measured in moles of photons per square meter per day (mol/m2/d). DLI is an important variable because the cumulative PPF delivered during a day has a profound effect on plant branching, rooting, stem thickness and flower number. The ideal DLI varies widely between species, cultivation environments, and at different growth stages. For example, to achieve peak production tomato crops require a DLI of 30-35, whereas a DLI of 13 is sufficient for vegetable seedling production, and propagation of cuttings needs only 4-6 mol/m2/d.
Lighting Technologies
Before the introduction of LED technology, high intensity discharge (HID) and fluorescent lamps had been used to grow plants. HID lighting systems, such as metal halide (MH), high-pressure sodium (HPS), are gas-discharge lamps that generate light by creating an electric arc between two electrodes. The fluorescent lamp is also a gas-discharge lamp but operates by exciting mercury vapors to produce ultraviolet light which is then down-converted by a phosphor coating. HPS lamps were the most commonly used greenhouse lights because of their relatively higher photon efficacy and better spectrum compared with metal halides or florescent lamps. HPS lamps better replicate the red and orange regions of the sunlight spectrum needed for flowering, allowing them to produce photosynthetically effective radiation for fruiting and flowering plants. Metal halide lamps have an output that emphasizes the blue part of the spectrum. This makes them better suited for vegetative growth and seedlings but less effective for fruiting and flowering. The spectrum of fluorescent lighting lacks the red emissions essential for carbohydrate synthesis, stem growth, flowering and germination, deeming them an incompetent light source for horticultural lighting.Conventional light sources suffer from various degrees of electrical inefficiency, whereas poor spectral power distribution (SPD) of these light sources compounds their overall inefficiency in horticultural applications. Not only a considerable portion of wavelengths is emitted outside the PAR region, these broad but fixed spectrum light sources have peaks in the green-yellow region of the light spectrum. This part of electromagnetic energy is wasted as it contributes little to plant photosynthesis.
Apart from spectral inefficiency, what limit the implementation of HID lamps in horticultural applications is that these lamps produce a large amount of infrared (IR) energy. Thermal radiation may affect the rate of plant development and damage plants. So MH and HPS light must be kept a safe minimum distance from the plants. Light sources that emit radiant heat do not fit into intracanopy and multi-layer lighting applications where light fixtures are required to be mounted at close proximity to plant canopy. In toplighting applications, as the distance is increased, the intensity of light from HID fixtures has to be increased to ensure a sufficient PPFD. Thus higher wattage lamps are required, which increases energy consumption and cost. Furthermore, greenhouses may require expensive HVAC systems or cooling equipment to remove the waste heat from HID lights.
The most recent evolution in horticulture lighting is solid state lighting based on LED technology. The use of LED technology has definitely revolutionized the way the horticulture industry operated years back and is opening up a whole new world of opportunities. An LED is a semiconductor device that can be configured to generate a light at a specified wavelength or a range of wavelengths, without energy waste outside of the PAR region. The spectral versatility of this technology allows horticulture lighting to target the active spectrum for any plant species or any growth stage of a plant. An extremely high spectral efficiency in conjunction with a high wall plug efficiency translates to unprecedented operation savings and accelerated payback. Long life cycle, maintenance-free operation and solid state durability drive a long return on investment (ROI). No filament, gas, or plasma is heated to produce light, absence of infrared radiation gives LEDs an exclusive advantage of offering maximum irradiance at close proximity to plant canopy. In particular, IR-free radiation enables innovative implementation of horticulture lighting such as intracanopy and multi-layer lighting.
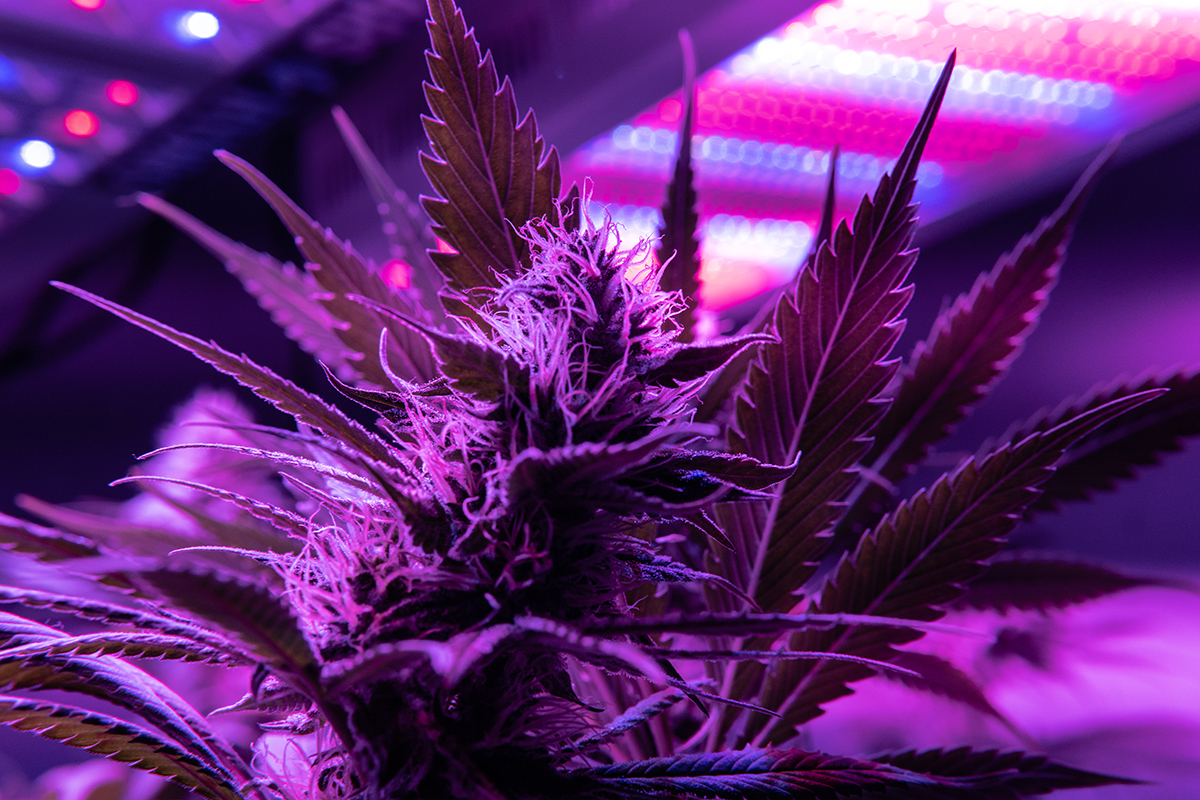
How LED Horticulture Lighting Works
An LED produces light when the diode is forward biased to cause electrons combine with holes inside the semiconductor junction. The semiconductor junction, also known as multiple quantum well (MQW) or active layer), is sandwiched between oppositely doped indium gallium nitride (InGaN) or aluminum indium gallium phosphide (AlInGaP) layers. Epitaxial layers for InGaN LEDs are grown on a sapphire, silicon carbide (SiC), or silicon substrate, whereas AlInGaP epitaxial layers are grown on a gallium arsenide (GaAs) or gallium phosphide (GaP) substrate. LEDs emit in narrow spectral bands. The wavelength or color of the light depend on the band gap energy of the semiconductor materials forming the active layer.Green, cyan, blue and royal blue LEDs are formed from InGaN semiconductor layers. AlInGaP LEDs are used to produce longer wavelength light such as red, red-orange and amber. InGaN blue LEDs are also used to fabricate full spectrum LEDs by coating a phosphor mixture onto the diode to down-convert a portion of the short wavelengths into longer wavelengths. An LED chip must be packaged to make itself a full-fledged device with electrical interfaces, thermal conduction path, mechanical support and environmental encapsulation. In horticulture lighting, multiple single-color LED packages are often used to provide additive color mixing for a dynamic light spectrum.
A high performing, high reliability LED system is a synergistic integration of thermal, electronic, mechanical, and optical components, with the purpose of unlocking the full potential of the LEDs. Given the reliability and lifecycle advantages of LEDs described above, it is not surprising that the most common failures of an LED grow light occur as a result of poor system engineering.
Among the engineering disciplines related to building LED systems, good thermal management is an indispensable prerequisite to the long lifespan and consistent performance of the LEDs. Thermal degradation is a major failure accelerator in LED lighting systems. Despite having a high energy conversion efficiency, LEDs converts typically around 40% of electrical power into light and the rest is wasted and dissipated as heat. LED grow lights are in most cases high power systems that incorporate a high density array of LEDs. The huge amount of waste heat produced by the LED array must be drawn away from the LED junction through a thermal path with thermal resistance reduced to a minimum. Failing to keep LED junction temperature at an optimal level will lead to thermal droop, photon flux depreciation, color shift, phosphor degradation, and premature failure.
The LED driver is the brain for an LED luminaire. It is critical to system reliability and performance, and can facilitate deployment of different technologies for advanced lighting control. LEDs are current driven devices and require a constant source of direct current (DC) during operation. A typical LED has a forward voltage between 1.5V and 4.5V below which no current will flow through the LED junction, while an excessively high forward voltage will cause the current to go above the maximum rating, resulting in overheating and the consequent performance degradation and shortened lifespan of the LED. As such, the fundamental role of an LED driver is to efficiently convert electrical power from AC mains to a DC load with outputs matched to the electrical characteristics of the LEDs. LED drivers also need to survive high transients and protect LEDs from damage caused by reverse bias, short-circuit, overcurrent events, etc.
In horticulture lighting, dynamic control of light intensity and spectrum is always appreciated to ensure that the right light recipe is delivered to plants. The ability to control the light output in a very dynamic way, including the quantity of light and the color of the light, is a significant advantage of LED lighting technology. Core to this ability is the dimming performance of the LED driver. The two most common dimming technologies are pulse-width modulation (PWM) and constant current reduction (CCR). PWM dimming is typically used in applications where accurate color mixing is needed for dynamic spectrum control, whereas CCR dimming is mostly used to adjust light intensity. PWM and CCR dimming circuitry can be controlled through a variety of dimmer-to-driver protocols such as 0-10V, DALI, and DMX.